In-situ density measurements have long been useful in process control applications from recycling nuclear fuel and refining oil to measuring beverage proof or degrees Brix (°Bx) sugar content of an aqueous solution. Density measurements can provide real-time determinations of concentration, blending ratio, fermentation, or oil API (American Petroleum Institute) gravity, which measures oil heaviness or lightness, etc. These can be used to adjust process variables and optimize throughput, quality, equipment and material utilization, and energy efficiency.
So it’s no surprise that densities of liquids, slurries and mixtures in vessels and pipes have been measured for many decades, and over those many years, density measurement technologies have been improved and refined. Today, in-process density measurements are commonly performed in vessels using servo gauges, differential pressure (DP), tuning fork instruments and sometimes radiometry. Flowing measurements can be done with radiometry and with Coriolis flowmeters.
Here’s an overview of real-time, in-process density measurement methods, with recent developments that might inspire additional or improved instrumentation.
Density fundamentals bubble up
Density is defined as mass per unit volume, typically grams per cubic centimeter (g/cc), but is often expressed in specialized units such as specific gravity (as a ratio to a standard such as water), API gravity (where 10 is the same as water), degrees Brix (specific gravity converted to sugar content), proof (calculated to reflect the percentage of ethanol), etc. The fact that the fundamental units of density—mass and volume—are simple (if not always easy) to measure makes density attractive as a direct and indirect measurement of attributes that might otherwise require complex and expensive analyzers.
Since most fluids expand and contract significantly with temperature changes—independent of the parameter of interest—the temperature of the fluid must often be taken into account when calculating properties. Measurements taken under significant pressure or vacuum may also require compensation for pressure-related density changes.
For example, for years, concentrations of acids in nuclear fuel recycling were measured by bubble tubes (Figure 1). By immersing a glass tube to a known distance below the surface of the acid solution in a vessel, providing a very small flow of pressurized gas to form intermittent bubbles, and measuring the gas pressure, engineers could determine the pressure exerted by the liquid above the bubble. With temperature and, if necessary, ambient pressure correction, they could measure the fluid density and, hence, acid concentration. Only the bubbler tube was exposed to the corrosive and possibly radioactive process—the gas pump/supply and pressure instrumentation could be placed at a distance.
[sidebar id =1]
Another way to weigh the volume of a liquid is by using a displacer—a float that doesn’t float. The displacer can be lowered using a servomotor and cable into and through the fluid, and the force required to support the float and cable can be measured to determine the weight of the displaced fluid.
“A servo gauge and displacer is often used to detect density interfaces in tank farm and crude oil applications,” says Gene Henry, business manager, U.S. level products, Endress+Hauser. The displacer can be lowered to detect the liquid level, then lowered through the liquid to detect interfaces between layers, including the level of any water in the bottom of the tank. “The gauge can report the density of each layer. If needed, the density can be corrected for temperature with the input from a multi-point temperature probe,” Henry says.
Hydrostatic and differential pressure
Pressures at known fluid depths are commonly used to measure level and/or density, either by a single measurement (hydrostatic) or by the difference between two measurements (differential pressure, or DP). Bear in mind that a pressure sensor at a depth can’t tell whether a pressure change is due to a level change or to a change in density—to determine one, the other must be held constant or compensated using an additional instrument.
“Hydrostatic level, using one pressure sensor, requires the fluid have a known density or specific gravity, so density changes introduce error,” says Jeff Brand, product manager, pressure, VEGA Americas. Adding a second sensor in the fluid at a known height above the first provides a DP measurement, and allows density to be calculated.
A common practice has been to use a single sensor (DP cell) that measures a difference in pressure, connected at two heights using impulse lines. These lines may be in the form of capillary tubes, sealed at the process end, and filled with special fluid. One variant is a directly-mounted seal at the bottom tap and a capillary to a single remote seal above it.
The required distance between the sensors depends on the density measurement range and the height of fluid above the sensors. “It’s complicated, but the results are very good once it’s set up,” Brand says. “As density changes due to the product or temperature, you can calculate that density and use it to get an accurate overall level.
“DP for density is usually used on open vessel applications such as flotation cells, mud pits and shakers on mud processing units where a vapor pressure would not interfere. Of course, both sensors must be immersed. In a pressurized tank, you can still calculate density but not level, as the instrument can’t tell the difference between a pressure and a level change.”
Instruments are available to mount on the outside of a tank, or through the top. “They’re immune to effects from agitation, foam or obstructions,” Brand adds.
The relatively low cost, simplicity and proven technology of DP has led to widespread use. “Everybody knows how to calibrate a pressure sensor,” says Nathan Stokes, product manager, DP level, Emerson Automation Solutions. “However, the span of DP for density is on the lower side. And if you use a DP cell, there are the challenges of impulse piping (leaks, plugging, fluid evaporation) and with remote seals, and error due to temperature changes affecting the density of the fill fluid.”
More recent developments include electronic systems to measure DP (Figure 2), with built-in calculations for density and density-compensated level outputs. “Now, electronic remote sensors eliminate the need for capillary tubes. Two separate sensors are mounted top and bottom to get a single 4-20 mA signal for DP,” says Stokes. “This system is accurate despite temperature changes. Its precision still varies with the application—the separation of the sensors—but it’s simple and cost-effective as a replacement for troublesome capillary systems. Eliminating the mechanical system increases reliability.”
[sidebar id =2]
Advances in sensors, seals, electronics, computing power and diagnostics are increasing precision, improving reliability and reducing maintenance. “Ultra performance class” transmitters offer precision to 0.025% of span, says Stokes. “Sensitive equipment can be fine when the process is running at status quo, but getting there can cause damage—an instrument can be out of spec from the beginning.” Overpressure protection allows significant overpressures without drift to withstand startup surges, slugs and spikes.
DP cell transmitters are being fitted with advanced diagnostics that can detect plugged impulse lines. “This is really helpful for those wet- and dry-leg applications where you get sediment buildup in the wet legs that can solidify, or get liquid buildup in the dry legs so sensitively is reduced,” says Wally Baker, content management, pressure, Emerson.
“Diagnostics also monitor transducers for overpressure, saturation or over-temperature conditions, so you can know when these conditions are present,” Baker says. “Advanced diagnostics can detect low voltages or brownouts, and diagnose power supply or water-in-conduit problems. That can be built right in via HART or wireless to give an alarm or notification.”
Stokes adds, “With wireless and simplicity of DP, you’re not cutting into lines—just the two taps—and not running wires, just using a battery that can last 10 years, so it’s easy to add a meter and get a density measurement.”
Tuning fork makes waves
Another technology that started with level detection and now does density is the tuning fork-based level switch. “Tuning fork detectors were developed for level, as the fork frequency in air is different than in liquid. When a difference of 20% is detected, they switch the output,” says Endress+Hauser's Henry. “But the density of the liquid affects the frequency, so tuning forks can be used to monitor density.”
Density also varies with temperature, so you also need a temperature probe and a density computer. And you can add a pressure sensor and input where needed. “The density computer output can be in degrees Brix or concentration rather than raw density,” Henry says. “It can indicate regular vs. high-test gasoline.”
The instruments are useful in batch operations, such as blending orange juice from concentrate, fermenting grape juice, or making apple vinegar. “You can tune the recipe with density, tell when the batch is fermented, or determine when to stop heating. You can control proof of rum,” Henry says.
Measuring density directly can have advantages in certain applications. “Acid concentration can be measured with conductivity, but many acids have a non-linear relationship—with a hump in the curve—so if it’s way off, you may not be able to tell,” Henry says. Density has a direct response with concentration.
Coriolis does all in one
While DP remains a fine way to measure density of liquids in vessels, Coriolis flowmeters have become an increasingly common way to determine densities of flowing fluids and mixtures. Capable of extremely precise and accurate mass flow measurement, the oscillation frequency of a Coriolis flowmeter's tubes is inversely proportional to the fluid density.
With the increasing sophistication and falling cost of digital signal processing, as well as lower costs for its demanding physical construction made possible by mass production, Coriolis flowmeter applications are on the rise. “The best Coriolis meter can measure to 0.0002 g/cc with no special adjustment required in the field,” says Nathan Hedrick, flow product marketing manager, Endress+Hauser. “They’re now commonly used in some cases to measure density, but many people take advantage of the multivariable capabilities. From a single instrument, you can get mass flow, density, temperature and even viscosity from some Coriolis meters.”
With available programming, “Density can be in the customer’s terms,” Hedrick says. “For example, concentration for food processing, degree API for the oil and gas industry, proof for liquor, or degrees Brix for beverages.” The output can give the percent concentration of blended fluids of known densities and expansion coefficients, such as glycol and water, or sugar and water. With temperature, that can be output as concentration or degrees Brix, and this can be done for any two fluids with enough difference in density," he says. “It’s not just flow, temperature and density—it’s a process analyzer, with output in industry-based units.
“A lab can be more accurate, but there’s not a much better inline measurement. By combining measurements in one instrument, the Coriolis meter kills two birds with one stone. You can save money or break even on capital cost, then save on maintenance.”
Radiometry: The nuclear option
Over the years, some of the most challenging in-situ density measurement problems have been solved using radiometric (also called nuclear or gamma) technology, where the intensity of gamma radiation from a source on one side of a process pipeline or vessel is measured using a detector on the other side. The intensity of transmitted radiation is related to the density of the process medium.
“The technology is beautiful because it’s completely impervious,” says Chris Willoughby, product manager, nuclear, VEGA Americas. “Unlike Coriolis, a bolt-on solution can be added without modifying the piping.” Radiometry has been used for many decades, and proven reliable in power plants, paper mills and metal ore processing. It’s suitable for a wide range of pipe sizes, wall thicknesses and density ranges, and is especially appropriate where pipe sizes are 3-4-in. and larger. It’s typically used for pipe diameters down to 2 in. and up to 36 in., including lined metal and plastic pipe.
“Radiometry is very prevalent in the mining industry, where they often combine a magnetic flowmeter and a density meter to get the mass flow of liquids and slurries,” says Henry. “Those non-intrusive technologies handle slurries well, without the tortured path of a Coriolis meter, which can lead to a lot of erosion.”
Radiometric methods are also used for level measurement, but usually those are typically not combined with density measurements. “Most nuclear level applications are on vessels that are too large to measure density directly—that’s typically limited to diameters of 3 ft or less,” says Willoughby. “But it can be done by inserting the source in a drywell with a detector on the outside. This is commonly done on separator processes in refineries using a multi-point density array. For example, in a desalter with an emulsion layer, we can put sources in a drywell, and use external detectors to generate a density profile to detect changes associated with an interface.”
The maximum diameter for radiometric methods depends on the strength of the radiation source and the sensitivity of the detector. Because the source is radioactive material, it's closely regulated worldwide, and requires a license to own, install, operate and service.
In the U.S., there are two levels of license: general and specific. The general license is limited to 270 millicuries (mCi) of Cesium-137 or 81 mCi of Cobalt-60 per source. “You just have to register the device with the state-approved body, or if there is none, the Nuclear Regulatory Commission (NRC),” Henry says. “It’s not that difficult, but you can’t install, remove or service the device.”
U.S.-specific licensing is more typical of world standards, and allows the license holder to use larger sources, and to install, remove and service devices. “Specific licensing requires a designated, trained and certified radiation safety officer (RSO) responsible for gamma devices in each plant,” Henry says.
Advances in detector technology have allowed lower-level sources to handle larger applications. “The old ion chamber detectors could handle an 18-in. pipe using 250 mCi Cesium-137 source. Now, using a scintillator tube with a crystal or PVT [polyvinyltoluene] detector, an 18-in. pipe can be done with 20 mCi. A lot more applications qualify for a general license,” Henry adds. A crystal detector is most sensitive, and can use the lowest-level source.
With the more sensitive detectors, “We can do more applications with Cesium, which has a half-life of 30 years,” Henry says. “Cobalt penetrates through more material and is used for the difficult level applications, but it has a half-life of 5.2 years, which means the source must be replaced more often. Today’s detectors can automatically compensate for this source decay.”
Like many other instrument technologies, signal processing and computing power are improving stability, Henry says. “Once they’re set up and operating, they hold their calibration. It’s not like in the past, with source decay and older detectors that would drift and need calibration twice a year. Now it’s once every couple of years to check and verify.”
Licensing can be an impediment, so “suppliers will offer assistance,” says Willoughby. They offer safety training, license services, consulting on safety programs and auditing. “They’ll review the user’s license before delivery, make sure it’s adequate and suggest revisions to make it more effective.”
As always, the best choice of in-process density measurement depends on the application—in some cases, it might make sense to add circulation just to use a Coriolis meter, but if the fluid is in a vessel, a tuning fork might be more appropriate. DP is often used on open vessels, radiometry on closed piping, and nuclear multi-point density arrays on pressurized processes.
Laboratory analysis may be more precise, but tuning forks, Coriolis meters, radiometric and differential pressure methods offer in-situ measurements. Whatever method is chosen, in-process density measurement can be a cost-effective way to improve process efficiency, throughput and quality.
[sidebar id =3]
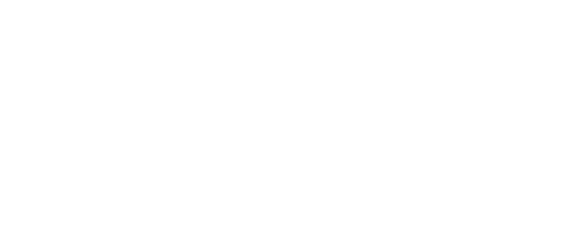
Leaders relevant to this article: