By Mark Clark, Alky Sulfur Operations Specialist, ConocoPhillips (Sweeny Refinery)
Steve West, Manager, Analytical and Temperature Product Development, Invensys Operations Management
1. Introduction
A vital process in petroleum refining is the synthesis of isooctane (i.e., octane) for blending in the dynamic gasoline pool. The most widely used process for octane production is HF Alkylation. In this process, HF (hydrogen fluoride; hydrofluoric acid; "acid") serves as a catalyst for the reaction of isobutane and C4 olefins to form octane or "alkylate." Prior to the development of the alkylation process, these low-molecular weight hydrocarbons were essentially waste. Alkylation turns these former waste products into the most valuable component of gasoline.
There are three main components in HF catalyst. HF is the principal component comprising about 90%. Water is usually present at around the 1% level, and the remainder is acid-soluble organics (ASO). Tight control of these constituent concentrations can save millions of dollars per year in an alkylation unit. Optimization requires monitoring the levels of all three components. In this paper, we describe our experience with a new, simple, safe and reliable system for monitoring HF catalyst composition at the ConocoPhillips refinery in Sweeny, Texas. The Invensys ACA.HF system has enabled optimization of the alkylation process for maximum profitability.
2. Control for Safety and Profitability
Safety and profitability of an alkylation unit depend on fine-tuning the controls and are maximized when the net consumption of HF is minimized. Operating at the lowest possible HF concentration, in regards to octane barrels, without risking an out-of-control phenomenon known as "acid runaway" is the optimal scenario—greatest output of octane barrels at minimum cost. If we can minimize the consumption of HF, the advantages ripple right down the supply chain. There are limitations on how much HF can be safely stored on site and it can take days to bring more in if unexpectedly needed. Accurate monitoring allows us to keep inventory low at the ConocoPhillips Sweeny refinery without risking a shortage.
Acid Runaway
The definition of a catalyst is: A substance that increases the rate of a chemical reaction without being consumed in the process. Optimal control of the HF acid regeneration operation can make a difference of millions of dollars in profit per year in octane barrels, or lost profit opportunities due to "off-color" alkylate product, non-utilized feed rates, and, worst of all, acid runaways. In acid runaway, HF’s role as a catalyst is compromised. This occurs when the HF concentration falls below a critical concentration threshold and begins participating as a reactant in undesired side reactions—reactions that consume HF and do not produce octane. Knowledge of HF and ASO concentrations are critical to avoiding this condition.
Water concentration is also critical. A small amount of water improves reaction efficiency, but too much water increases the corrosiveness of the catalyst.
In order to avoid acid runaway, an HF concentration margin must be maintained over and above the critical concentration. Obviously, uncertainty around the HF and ASO concentrations at any time requires us to maintain a greater margin and results in poorer efficiency. Accurate, real-time concentration data allows us to run at a tight yet safe margin with confidence.
HF Alkylation Process HF is not consumed; it is recycled.
3. Existing Monitoring and Control Strategies
A prerequisite for control of a process is knowledge of the key process parameters, in this case, the concentrations of HF, ASO and water in the reaction mixture. Two monitoring strategies can be found in the industry today: grab-sample extraction for laboratory analysis and online analysis.
Grab Sampling / Lab Analysis
We have nothing but the highest regard for the chemists and technicians who perform chemical analyses at our ConocoPhillips refinery laboratories. In spite of the challenges described below, the skills and training of these individuals have ensured safe, efficient operation of alkylation units for decades. Nevertheless, the disadvantages of grab sampling and lab analysis of HF cannot be overemphasized. These disadvantages arise on two fronts: safety and accuracy.
The hazardousness of HF is extreme. Not only is it severely toxic through ingestion, inhalation, or contact with skin, it is highly volatile, permeating, and corrosive. Grab samples must be taken in sealed, Monel sampling bombs by personnel wearing full protective gear and respiratory apparatus. Personnel with special training must carry out analyses in a designated, segregated laboratory area with safety showers present in the lab and along the transport route.
Analytical Challenges
HF catalyst is a reactive mixture—removing the grab sample from the process does not stop the reaction. In other words, the clock is ticking on how representative the grab sample is of the process. Removal of the sample from the bomb, and all subsequent handling must be carried out anaerobically using materials and instruments that can withstand exposure to HF. This precludes the use of ordinary laboratory glassware, most metals and plastics. No matter how efficient the analyst or accurate the results, uncertainty grows as time elapses between sampling and the return of the results. This time is at best hours, often a whole day.
Online Analysis
The composition of HF catalyst can be determined by the technique known as Fourier-Transform, Near-IR Spectroscopy (FTNIR). This powerful tool analyzes the near-IR absorption spectrum of a mixture and breaks it down into contributions from its components. Using a pre-developed chemometric model, concentration values for target components can then be derived from the IR spectrum. In the 1990s, online FTNIR instrumentation was developed for HF alkylation analysis. Moving the analysis from the lab to the process proved enormously useful. In addition to the advantages that can be enumerated by turning the above-mentioned disadvantages of grab sampling and lab analysis upside down, real-time online analysis allows us to "skate closer to the edge", in other words to narrow the control margin and realize higher efficiency.
4. The Invensys ACA.HF Solution
Although the online FTNIR brought a completely new level of control to an alkylation unit operator, the up-front and ongoing cost of the instrumentation leaves many refineries continuing to rely on lab analysis. The desire to provide the advantages of real-time, online monitoring at a lower cost led to a ConocoPhillips collaboration with Invensys on the development of the ACA.HF system for HF catalyst monitoring. In addition to lower up-front cost and a reduction in maintenance, goals in the effort were simplicity, robustness and the use of proven process analytical hardware.
Analytical Strategy
Products from the Invensys Foxboro Measurements & Instruments (M&I) brand have been used for decades in refineries and many other industries. Simple, robust analytical measurements have an established track record in the characterization of 2-component solutions. Common examples are the use of a density measurement to determine the antifreeze content of automobile engine coolant, or refractive index to measure the sugar content of wine. To characterize binary solutions, one measurement that varies uniquely with solution composition is required, because determination of one component allows the other to be inferred as the remainder to make up a total of 100%. Analogously, a ternary solution can be characterized by two measurements, so long as they vary uniquely with the concentrations of the constituents.
The dissolution products are ionized species, which make the solution conductive. In binary HF/water solutions, the concentration of conductive species and therefore the conductivity is determined by the water concentration (see accompanying figure).
Fortuitously, the third major constituent of HF alkylation catalyst, ASO, dissolves without the creation of ions. This means that a conductivity measurement is an uncompromised predictor of water concentration in the reaction mixture.
Conductivity is a highly developed measurement technique with a long legacy in process control. In the alkylation application, an electrodeless, non-contacting conductivity sensor was selected. In this configuration, the process passes through an unobstructed bore of inert material—a simple pipe. External to the bore, a coil called a "drive toroid" generates an oscillating magnetic field, which induces an alternating current in the process fluid. That current in turn generates an oscillating signal in a second external coil called a "sense toroid". The signal in the sense toroid is proportional to process fluid conductivity which is proportional to the water concentration.
Conductivity provides a handle on water, then a second measurement—density—is used to determine the HF and ASO concentrations. Light hydrocarbons have densities as low as 0.6 g/cc, whereas the density of pure HF is very nearly 1.0 g/cc, almost exactly the same density as water. Thus, the relative concentrations of HF and ASO affect the density of the alkylation mixture while water does not. Just as density may be used to determine the composition of a binary mixture, for example, ethanol and water as shown in the accompanying figure, it serves in the ACA.HF system to determine the concentrations of either HF or ASO.
Second-Order Effects
Accomplishment of the HF catalyst analysis is not quite as simple as described. Two important aspects were not included for simplicity of explanation: the effect of temperature, and the second-order influence of interactions that may occur between the ASO and water.
All species in a solution affect each other directly or indirectly. In the present case, the assumption that conductivity can predict water content and that density can predict HF/ASO content in a ternary solution turned out to be extremely powerful (see figure below). However, corrections for temperature and interactions between the components were built into the ACA.HF model as refinements.
Model
A chemometric model was developed by recording conductivity (Cond), flow (Flow), pressure (P), density (D), and temperature (T) of HF alkylation catalyst in an operating alkylation unit using the online ACA.HF system. The ACA.HF data set was time-correlated to %HF, %H2O, and %ASO from plant records over a period of four months. Using multivariate regression techniques, the data was fit to equations that predicted %H2O and %HF as functions1 of conductivity, density and temperature:
%H2O = ƒ1(Cond) +ƒ1 (Dens) + ƒ1 (Temp)
%HF = ƒ2 (Cond) +ƒ2 (Dens) + ƒ2 (Temp)
ASO was then calculated2,3:
%ASO = 100 - %HF - %H2O
The graphs to the right illustrate the tight agreement between ACA.HF and online FTNIR analyses, demonstrating that the simpler, more robust ACA.HF sensing strategy can deliver high-quality results.
Hardware
Referring to the system diagram below, the Invensys system consists of a sampling panel located in the hazardous area. All fluid-handling components, sensors and signal transmitters are located at the sampling panel. Signals are transmitted to an Invensys Eurotherm DCS and Invensys Wonderware HMI in the safe area.
¹ Proprietary functions.
² Because of refinery precedent, %ASO was determined by subtraction after calculating %H2O and %HF from the regression model. Determining %ASO by regression and %HF by subtraction is mathematically equivalent.
³ In keeping with industry precedent, results are actually reported on the basis of a 94% rather than 100% total. This assumes a 6% loading of entrained isobutane.
Advantages of ACA.HF over FTNIR
Although an FTNIR spectrometer is an extremely powerful analytical instrument, its adaptation to online operation in an alkylation process is a complex undertaking whereas conductivity and density sensors are extremely simple and reliable—essentially just lengths of pipe. Their use in HF media has long been established. ACA.HF achieves further simplification through elimination of the need to control sample temperature, and through direct installation into the fast loop. Analysis of a continuously flowing sample system obviates the need for a complex sample handling system with a frequently cycling shutoff valves that require maintenance and periodic replacement. ACA.HF outputs its own set of diagnostic information—flow rate, pressure, and temperature. The cost of an ACA.HF system is about half that of online FTNIR and in just over one year at the ConocoPhillips Sweeny refinery, there has been no required maintenance. It was commissioned in less than 2 days, requiring no special skills to install and operate that are not found in every refinery. The components are laid out on an open panel requiring no inert gas purge or HF vapor detection.
Real-time, accurate concentration data helps keep HF down and ASO up. The trouble with FTNIR at high ASO is fouling of the cuvette window, which requires the FTNIR unit to be shut down for cleaning. The ACA.HF system does not have these types of problems.
5. Summary
The advantages of online alkylation process monitoring are clear. Fine-tuning the concentrations of the key parameters—HF, ASO and water—improves profitability and safety. The Invensys ACA.HF Alkylation Solution has demonstrated at the ConocoPhillips Sweeny refinery that continuous monitoring can be achieved with lower installed cost, less maintenance, and higher reliability than ever before. At Sweeny, the ACA.HF system quickly became depended upon as the workhorse for control of the HF alkylation unit.
©2010 Invensys Systems, Inc. All rights reserved. Avantis, Eurotherm, Foxboro, InFusion, Invensys, SimSci-Esscor, Skelta, Triconex, Validation Technologies and Wonderware are trademarks of Invensys plc., its subsidiaries and affiliated companies. All other product names are the trademarks of their respective holders. rel. 08/10 PN FX-0111
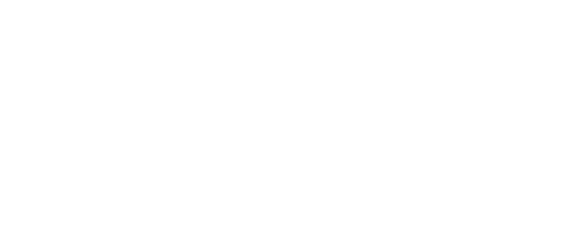
Leaders relevant to this article: