Whether you're brewing beer, creating clean water from wastewater, or producing pharmaceuticals, flexibility is what you need when you're controlling fermentation. Along with effective coordination, flexibilty is crucial when you want to minimize waste of material and maximize product quality throughout all phases of a typical fermentation process (that is, sterilization followed by cool down and then fermentation).This article focuses on the factors that impact dissolved oxygen (DO2) and how to simplify and maintain tight, responsive control.Dissolved oxygen is an important factor because it is a regulator of metabolic activity. Nutrient issues aside, the amount of dissolved oxygen available to the growing culture can easily become a growth-limiting factor, especially when conducting high cell density fermentations.When the Waksman Institute of Microbiology at Rutgers (Piscataway, N.J.) needed to improve process control in its fermentation applications, its staff conferred with the process control engineers at ControlSoft, Inc. (Highland Heights, Ohio), and outlined a specific goal: faster and tighter control of DO2 to process requirements. To accomplish this and to achieve the highest possible product quality, we knew that we needed a flexible control strategy. Of course, we also wanted to save money wherever possible. ControlSoft’s engineers recommended its MANTRA Advanced Process Control system.Change is GoodThe ability to change control strategy is critical for slow processes such as fermentation because of the mechanical problems that can occur during the lengthy phases of the process. At Waksman, the typical key parameters are temperature, pressure, pH and DO2, enabling the growth of both anaerobes and organisms requiring high oxygen transfer. Waksman needed flexibility to provide its process engineers with the opportunity to save a batch in spite of faulty conditions that may develop over during the process. A few months after our first meeting the new control software was ready to be added to our PC network.
The Application
A typical fermenter is shown in the Figure. The circulation pump runs hot or cold water through the jacket to maintain temperature in the vessel (in this case, 121° C for sterilization or 37° C for fermentation). Sterilized air is run through a sparger into the media in the fermenter (typically proteins, sugar, water and the culture), and then is released through the exhaust piping. The air exhaust valve maintains pressure in the fermenter. The sparger control valve maintains the desired flow of air. Agitator speed is maintained via a motor and variable-frequency drive (VFD).Fermenter Control PointsDissolved oxygen concentration is affected by a numberof factors: agitator speed, air supply and vessel pressure. Thequestion is: How best to achieve tight control of the variables?Answer: Model-Predictive-coordinated control.DO2 content is monitored via a side-entry probe and controlled by adjusting either agitator speed, air flow or air pressure in the bioreactor. The addition of acid, base, nutrient or antifoam is controlled using solenoid (On/Off) valves to inject proper solutions from pressurized addition tanks.
Three Phases
The three basic phases for each fermentation run are heat-up, cool down after sterilization, and fermentation. In Phase 1 the bioreactor is filled with the media (nutrients and water) and sealed. Then steam is injected into the jacket, and temperature is raised to 121° C. For proper sterilization, the temperature must stay at 121° C for a predetermined period. During this time the pressure in the bioreactor increases to about 15-20 psi due to steam generated from the water in the media. The combination of temperature, pressure and time sterilize the media and bioreactor system.During Phase 2, the bioreactor is cooled down to a specified operating temperature. To achieve this, cooling water is injected into the jacket. During the cooling phase, pressure in the bioreactor drops rapidly due to steam condensation. At this point, sterile air is introduced into the bioreactor to compensate for the pressure drop. This is necessary to avoid creating a negative pressure and to maintain the liquid level in the bioreactor.In Phase 3, fermentation starts when the bioreactor is inoculated. The culture starts growing, consuming nutrients and oxygen, producing heat, and in some cases, acids and other byproducts that may be harmful to the culture. Maintaining correct growth conditions (temperature, pressure, pH and DO2 content) is essential for successful completion of the fermentation process. The reaction may last from a few hours to days, but typically it does not last longer than one week. Samples are periodically taken to observe microbial growth and assay for products and various nutrients. When the fermentation is complete, the bioreactor is usually chilled, followed by myriad downstream steps that are required to isolate and purify the fermentation product(s).DO2 Concentration
DO2 concentration is affected by the speed of the agitator, by air supply, and by the pressure of the vessel. The crucial question is: When and how should these factors be used to best achieve the tightest control of DO2 in a particular application? Waksman needed the ability to change strategy on the fly, at any point of the fermenting process. The new control system gave us that ability by helping us control critical variables including:Agitator RPM: If a motor with a variable frequency drive is controlling the speed of the agitator, a PID controller can regulate the motor's speed, which can then be used to increase the DO2. Alternatively, the agitator can be set at a desired constant speed.Air Flow: Air flow is effective in directly increasing DO2. The air flow loop is a PID loop whose purpose is to properly maintain the air flow setpoint. Pressure Control: The pressure of the vessel is regulated by a PID loop. Constant pressure will be maintained normally, but the pressure can be activated for DO2 control if the agitator and/or air flow are at maximum limits.Waksman’s engineers encountered some problems with the data highway link between the computer and the external CPU. Data sampling time and heavy-duty tasking led to freeze-ups in the CPU. Eventually, a more distributed system was designed and implemented. The problem continued, albeit with less frequency. With a redesign in the external CPU software, the freeze-up problem should be resolved.During the first six months of operation with the new control system we experimented with PID values in particular. PID control loops and an advanced model-predictive coordinated controller (CC) form the heart of the control system logic. The best solution we found was using the new system’s CC block to keep DO2 content to desired levels.Model-Predictive Coordinated Control
The CC block controls a single process variable by manipulating three different controller outputs. The goal of the CC block is three-fold: reject any disturbances to the process, respond quickly to setpoint changes, and optimize the available controller outputs during steady-state control. The CC block meets these goals by using two independent lists named "Active" and "Target." The Active List tells the CC block which controller output device is the strongest and most effective. Since agitator speed, air flow and pressure affect the DO2, the CC is used as the main controller to control DO2. A typical control strategy requires the CC to use the agitator as primary control to maintain DO2, followed by air flow and, finally, pressure. Each successive controller is activated when maximum setpoint operating values are obtained in the preceding controller. RPM, air flow and pressure can also be activated as singular, individual DO2 controllers. The Target List is used by the CC block to control the long-term steady-state value of each controller output. Simultaneously with the process variable growing towards its desired setpoint, the CC block drives all available controller outputs to their target values without upsetting the process. This means that target outputs can be set to the desired optimal and/or effective control conditions. If faulty conditions threaten to spoil a batch, strategies can be modified at any point during the fermenting process.Ultimately, using CC has allowed Waksman the flexibility needed for faster and tighter control of DO2 levels in relation to process requirements, and helped the facility better accommodate a larger number of clients and a more varied client base.Ken Callanan is Supervisor, Cell & Cell Products Fermentation at Waksman Institute of Microbiology, Rutgers University.Latest from Asset Management
Latest from Asset Management
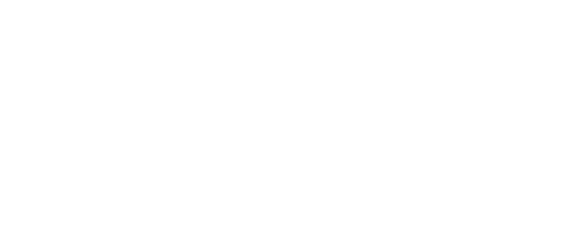
Leaders relevant to this article: