This article was printed in CONTROL's November 2009 edition.
Controlling and optimizing a process—any process—requires the full understanding of the characteristics of that process. Just as elephants have "personality," mechanical pump stations also have their own. Therefore, before showing you how to control and optimize pumping stations, first I will describe the pumping process itself.
The Process of Pumping
Pumping is a very energy-intensive process. The lifetime operating electric energy cost of a pumping station is about 100 times its first cost. In this series of articles, I will show that 20% to 60% of the pumping energy can be saved by optimization. This is significant because centrifugal pumps consume between 25% and 60% of the electrical energy consumed by the various motors in various industries. For example, the numbers for three important industries are as follows: petroleum, 59%; paper, 31%; and chemical, 26%.
The main components of all pumping stations are the electric motors and the pumps. The efficiency of old motors is in the range of 60% to 80%. The range for new pumps is 81% to 85%. The efficiency of old, constant-speed pumps is in the range of 40% to 60%. The range for new pumps is 61% to 70%. In addition to these losses, in certain applications such as in agricultural, power transmission losses can also be significant (about 10%).
In an article in the June 2003 issue of Paper Age (Pemberton, M., "Strategies for Optimizing Pump Efficiency and LLC Performance"), the U.S. Dept. of Energy says the replacement of old motors and pumps with newer designs does not result in large savings—1% to 2% for pumps and 2% to 3% for motors. Larger savings can be obtained by changing the operating modes of the pumping stations. These savings are as follows:
- Up to 20% by stabilizing flows using surge vessels,
- Up to 30% by matching variable loads with multiple instead of constant-speed pumps,
- Up to 60% by replacing control valves with variable-speed pumps.
The elephant displays an efficient pump design.
In this series, I will concentrate on control and optimization strategies that can provide up to 60% saving by eliminating all control valves and, therefore, all valve-throttling related energy waste. The goal of pumping station optimization is to introduce only the energy that is needed to transport the fluid, but no more. The elimination of energy waste, while guaranteeing accurate supply-demand matching, will not only lower operating costs, but also reduce maintenance. The full optimization of pumping stations—including automatic start-up and shutdown—will also eliminate human errors and increase operating safety.The "Personality" of the Pump
A pump is a liquid transportation device which must develop enough pressure to overcome the hydrostatic and frictional resistance of the process as it delivers the required fluid. These resistance components are unique characteristics of the process being served, and can be described by system curves. The system curve of a process relates the pressure (head) required to the generated flow rate.
The characteristics of the system that is served by a pump or pumping station can be represented by a head-capacity system curve (Figure 1). The head at any one flow capacity is the sum of the static and the friction heads. The static head does not vary with flow rate. It is only a function of the elevation or back pressure against which the pump is operating. The friction losses are related to the square of the flow, and represent the resistance to the flow caused by pipe and equipment friction.
A generalized equation describing the system curve of a process is:
P = H + Ff(Qx)
Figure 1. The system curve crosses the pump curve at the operating point.
where P is the required pump discharge pressure; H is the static or elevation head; Ff is the friction factor; Q is the flow rate; and x is an exponent that varies between 1.7 and 2.0 (usually 2.0 is used).
Figure 1 illustrates the typical pump curves of a single impeller pump. It shows both the head-capacity curve of a centrifugal pump and the system curve of a process. The operating point is the point at which the pump and system curves cross. If the process flow is controlled by a control valve, this generates a new system curve because the pressure drop through the valve is added to the basic system curve. This friction loss added by the valve is a source of energy waste, which can be eliminated by not using valves, but modulating the pump speed.
In addtion, at www.controlglobal.com/LL0911.html, a 3-D plot (a = pressure, b = flow, c = speed), where the system curves form one surface (surface A) and the pump curves form another surface (surface B) is shown. The intersection of these surfaces represents the operating line of the variable speed pump.
In the past, control valves were used to control the flow, because most AC electric motors were constant-speed devices. Therefore, in the past, variable-speed control was only common when steam turbine drives were used. Today, with the advent of pulse-width modulated (PWM) adjustable-speed drives using sensor-less, flux-vector control, adjustable-speed (AS) pumping is common in all applications.
Savings Potential
The amount of energy savings resulting from switching to variable-speed pumping is a function of the system curve’s shape. All system head curves are parabolas, but they differ in their steepness, which is a function of the ratio of static head to friction drop of the process served. The system curve is flat if most of the pumping energy is needed to overcome elevation or backpressure (static head), while it is steep if most of the pumping energy is used to overcome friction. Therefore, in mostly friction systems, the savings will be greater.
Cavitation
When optimizing pumping stations, another important consideration is cavitation. Cavitation is caused when the pumped liquid flashes inside the pump when the pressure on the suction side drops below the vapor pressure of the pumped liquid. This vaporization can occur either because of a rise in vapor pressure due to increased temperature, or because of a drop in suction pressure, caused by increased pipe friction. In either case, when vapors are present, as the spinning impeller throws the liquid and vapor outward, the vapor bubbles collapse as the pressure rises above the vapor pressure. This collapsing results in erosion. The erosion is most likely to occur at the impeller, because, as the bubbles collide with a solid surface, they suddenly release their energy of condensation by "implosions."
This sudden release of large amounts of energy causes the generation of high-intensity sound waves, which tend to intensify in the summer, when the ambient temperatures are higher. This characteristic sound of cavitation resembles the sound of gravel in a concrete mixer.
One might try to eliminate or minimize cavitation by removing restrictions at the pump suction. If the pump cavitates at high flows, a second pump should be started at a lower flow. If cavitation occurs at low flows, one might turn off the pump. An extreme option is to inject a compressible gas into the impeller. This reduces pump efficiency and capacity, but it can eliminate cavitation.
In the next installment, I will continue to describe the "personality" of the process of pumping and will start explaining its control and optimization.
Béla Lipták, PE, control consultant, is also editor of the Instrument Engineers’ Handbook. He can be reached at [email protected].
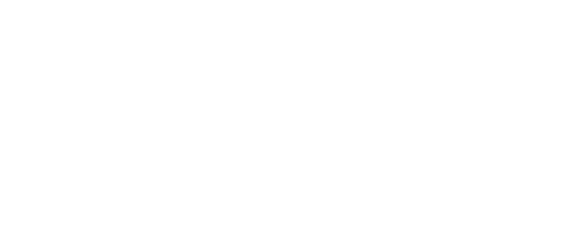
Leaders relevant to this article: